CSD in Action: Understanding Hydrogen and Halogen-bond Geometries
Here we highlight a paper where the Cambridge Structural Database (CSD) was used to understand differences in hydrogen and halogen-bond geometries of 5-membered heterocyclic rings.
You will learn about:
- The structural investigation that the team performed;
- How they used experimental data and computational analysis to understand the differences between the geometries of the two bonds and what causes these differences;
- How this analysis can help to develop compounds that can selectively form hydrogen or halogen bonds and direct the formation of specific topologies.
Why?
Halogen and hydrogen bonds are important intermolecular forces that occur in molecular systems. Despite having shown some similar properties, these bonds usually exhibit different geometries (Figure 1 a and b).
A class of compounds in which the geometries around hydrogen or halogen bond donors are expected to be similar are hydantoins (Figure 1 c).
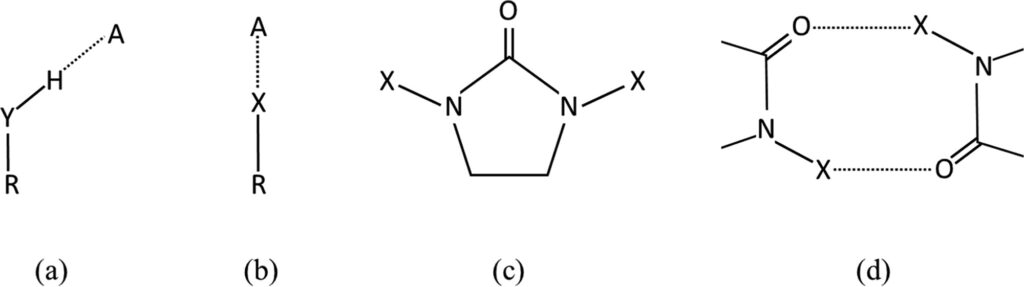
From a search of the CSD the scientist identified that halohydantoins (hydantoins with X = Cl, Br, I) are quite rare, with only 23 crystal structures reported in the database. All of them form halogen bonds with pyridine derivatives in cocrystals, or with the O=C group of a neighbouring halohydantoin.
Compared to halohydantoins, hydantoin derivatives (having X = H) are instead more common, with 262 structures in the CSD, all forming hydrogen bonds that involve the N-H moiety. Interestingly, while the most common synthon in these structures is the hydrogen-bonded ring, R22(8), reported in Figure 1 d, only one of the halohydantoins (CSD Entry: YOPJUJ) exhibits a similar motif.
Aiming to understand the nature of these differences and get insights that can help to direct the formation of specific topologies, the scientists investigated the hydrogen and halogen bond behaviours of hydantoins and other five-membered rings containing similar moieties.
How?
The Halogen Bond Investigation
To investigate the halogen bond in halohydantoin-like structures, the team started by expanding the search of the CSD to five membered rings including the fragment reported in Figure 2, with X = Cl, Br, or I.
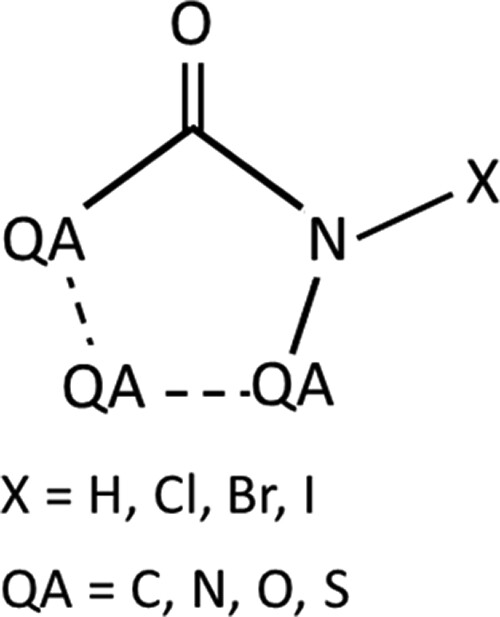
A total of 140 unique structures were found, all exhibiting interactions involving the N-X moiety.
In multicomponent systems, the most common interactions seen were the halogen bonds N-X···N (most of which involved pyridine derivatives) or N-X···OpyrNox (where OpyrNox are pyridine N-oxide derivatives).
In single component systems, 82% of the crystals formed a N-X···O=C halogen bond, and only 1 of the 140 structures, CSD Entry: YOPJUJ (Figure 3), formed an R22(8) halogen-bonded ring.
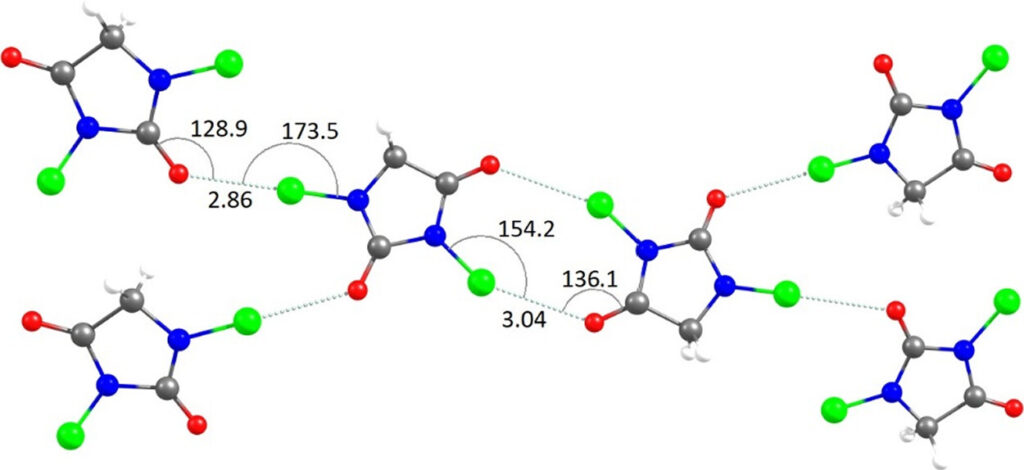
The Interaction Energies
To further investigate the system, the team performed computational studies on simple unsubstituted hydantoins (Figure 1 c) with X = H, Cl, Br, I (named 1H, 1Cl, 1Br and 1I).
For all the hydantoins, the structures of the calculated optimized geometries of dimers exhibited the R22(8) hydrogen- or halogen-bonded motif.
Looking at the interaction energies for the homodimers 1X···1X reported in Table 1, it can be seen that the 1H···1H interactions are stronger than those between dimers of halohydantoins and that 1I forms the strongest halogen bond. However, it is interesting to note that halohydantoins are capable to form interactions with Cl– ions and pyridine molecules that are stronger than the ones that involve 1H.
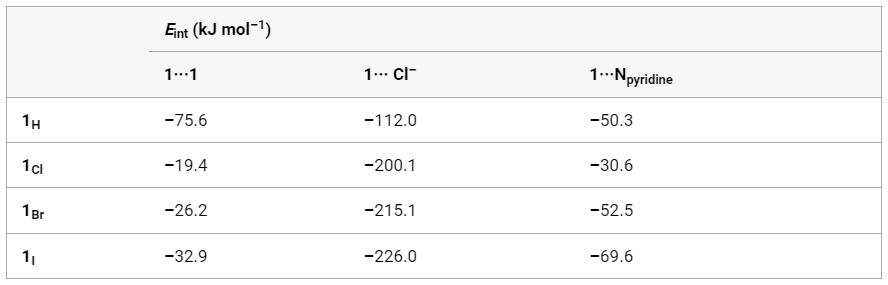
These observations allowed the scientists to conclude that the reason why the R22(8) halogen-bonded motif is rarely seen experimentally is not due to the relative strengths of hydrogen vs halogen bonding, as the latter are usually stronger, but rather to other aspects that still needed to be investigated.
The R22(8) Ring Geometries: an Analysis of the CSD
The team continued with a search of the CSD for five membered rings including the fragment reported in Figure 2, with X = H.
A total of 4531 unique structures were found, of which 4353 exhibited interactions involving the N-X moiety. Out of the 3354 unique structures that contained the N-H···O=C- interactions, 1824 exhibited the R22(8) hydrogen-bonded motif.
The scientists used this search and the search performed above for halohydantoin-like structures to look at the distribution of bond angles. They discovered that a very narrow distribution of values is seen when the N-H···O=C interactions are involved in a R22(8) hydrogen-bonded ring, both for the N-H···O angle (close to 180°) and the H···O=C angle (mean 121(7)°, Figure 4).
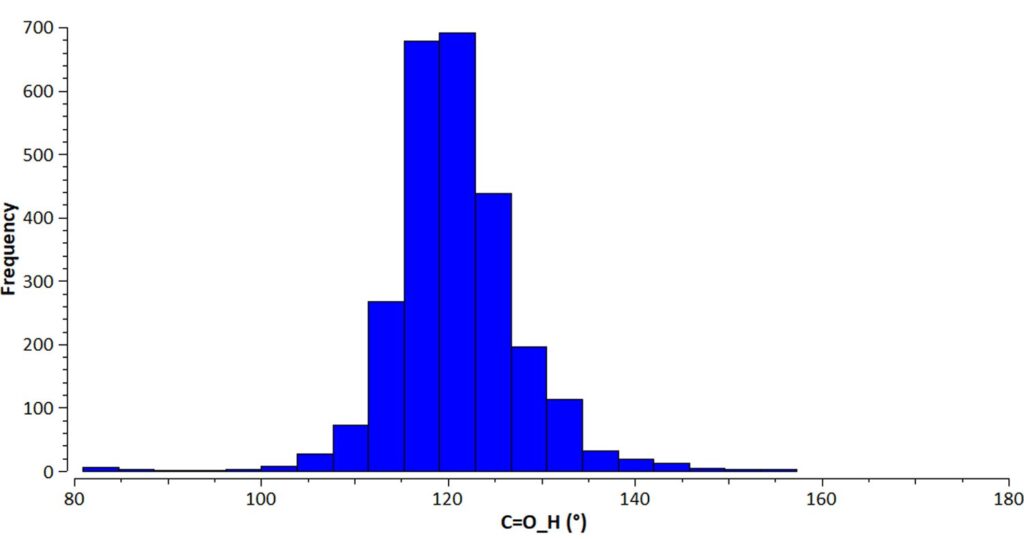
Halohydantoin-like structures involving the N-X···O=C halogen bond, exhibited instead less linear N-X···O angles and larger X···O=C angles (close to 140°).
To better understand if the lack of experimental structures involving halogen-bonded rings was related to a greater strain within the ring, the scientists performed a computational analysis of the angular dependence of the N-X···O=C interactions.
The Angular Dependence
To start with, the team confirmed the results seen experimentally for the ideal angles within a ring through computational calculations and proved that it is less energetically advantageous to form a halogen-bonded ring.
As a second step, the scientists performed an experiment on pairs of hydantoin molecules forming the idealized geometries: N-X···O angles of 180°, X···O=C angles of 120° and DXO distances of the optimized structures (Figure 5).
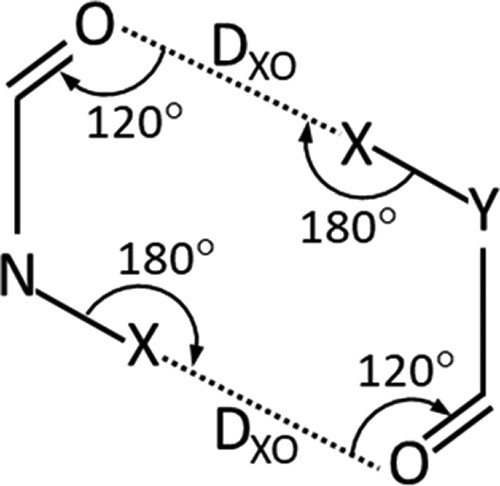
Reprinted with permission from Crystal Growth & Design 2024, 24, 2, 859-870
It was observed that in the idealized geometries, the dimers forming hydrogen bond were stabilized of more than 60 kJ mol-1, while all the halogen-bonded dimers were destabilized. A deeper analysis showed also that the latter exhibited close contacts between the halogen atoms that led to a very high repulsion, and that the change of N-X···O angles relieved this strain.
Conclusions
In this work, the scientists investigated the hydrogen and halogen bond behaviours of hydantoins and other five-membered rings containing similar moieties.
The team accessed the wealth of structural data deposited in the CSD at multiple stages of their research, and searched for the most likely bond angles, lengths and geometries found in hydantoins and derivatives.
The observations derived from the experimental data in the CSD, combined with computational calculations, allowed the scientists to identify that the different geometry found in halohydantoins was caused by repulsive X···X contacts in the halogen-bonded rings.
Next Steps
To discuss further and/or request a demo with one of our scientists, please contact us via this form or .
Read the full paper here: Crystal Growth & Design 2024, 24, 2, 859-870.